Towards nuclear fusion: Certifying the suitability of materials for fusion
The material requirements for a fusion power plant are very challenging
In previous issues we have seen that the most promising reaction for the first generation of fusion power plants is the deuterium-tritium reaction. We also know that this reaction generates neutrons, with energy of 14.1 MeV, which will strike the reactor vessel and its internal components. This neutron flux will cause structural damage due to material degradation —usually quantified in terms of “displacements per atom” (dpa), a characteristic magnitude for quantifying neutron-induced decay that indicates the average number of atoms that have moved to interstitial positions in the bombarded material. A commercial fusion reactor will require materials capable of withstanding 150 dpa, a quantity whose consequences are far from being studied. Therefore, in order to design a feasible reactor (let alone for the relevant regulatory body to license its installation), it is urgent to understand what materials can be used and how their mechanical properties will vary during their lifetime. And this is where IFMIF comes in, which is a unique tool for learning this. But let’s not get ahead of ourselves.
The need for a neutron source Conventional fission reactors produce neutrons with an average energy of 1 or 2 MeV, which are therefore inadequate to meet the requirements of fusion materials. Spallation neutron sources are also inadequate. Their energy spectrum is certainly broader, but they tend to generate light nuclei that unsatisfactorily affect the properties of the alloys to be certified. On the other hand, ion implantation facilities offer an irradiation volume that is not sufficient for standardized testing of mechanical properties. In short, the sensitivity of materials depends specifically on the irradiation and temperature conditions. Thus, a neutron source comparable to those produced in a fusion reactor is needed to certify its suitability for this stressful environment. And IFMIF’s deuterium-lithium reaction will be able to provide neutrons with an appropriate energy spectrum. |
About fifteen years ago, a debate was opened in the science and technology fusion community: testing materials directly in the ITER machine (which implied changing the first reactor wall and thus keeping the system shut down for several years)? Building a DEMO prototype to test everything simultaneously and replace only what degraded (a trial-and-error option)? Setting up a machine to simulate the neutron flux of a commercial fusion reactor, and testing samples of materials for as long as necessary, without interfering with the physics and fusion technology programs, which would evolve in parallel?
The latter alternative, proposed in the mid-1990s, is the so-called IFMIF project (International Fusion Materials Irradiation Facility). The results obtained through IFMIF, together with those of ITER will make it possible to design DEMO: a demonstration thermoelectric fusion power plant that will be the mould for the first generation of commercial thermonuclear fusion power plants. The IFMIF project would also have other advantages, since it could not only continue testing new materials for the next generation of fusion power plants, but it would also be a very useful source of neutrons for other scientific and technological uses.
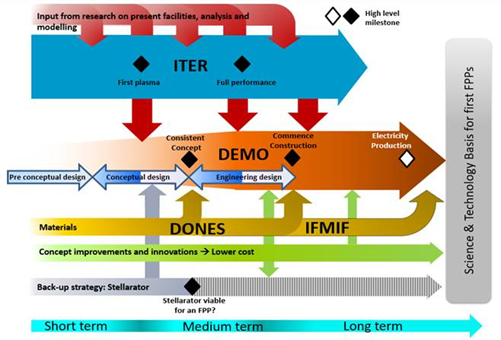
IFMIF will thus be a source of neutrons with an energy spectrum analogous to those produced in a deuterium-tritium-fuelled nuclear fusion reactor, which will make it possible to certify the suitability of candidate materials for fusion power plants.
IFMIF an essential step in the development of fusion
The IFMIF project is a scientific research program launched in 1994. It is international in nature, involving Japan, the European Union, the United States and Russia, and is managed by the International Atomic Energy Agency. Basically, IFMIF consists of two parallel deuteron accelerators bombarding a 20 x 5 cm liquid lithium circulating target to produce a high flux of neutrons. The beam energy (40 MeV) and the current of the two accelerators (2 x 125 mA) will be adjusted to maximize the neutron flux produced with an energy spectrum similar to those that will hit the first wall of a commercial fusion reactor. Successful validation of the small sample testing technique will allow full mechanical characterization of the candidate materials and thus understand their degradation process. This will lead to the design of components more capable of withstanding neutron bombardment over the life of a commercial fusion reactor.

IFMIF/EVEDA, prelude of IFMIF
In the 1990s, a pre-design of IFMIF was already proposed, consisting of three main facilities: two accelerators, a lithium target and a test cell. However, as doubts arose about the feasibility of IFMIF, because it was questionable whether the required high deuteron current could be controlled, IFMIF/EVEDA (Engineering Validation and Engineering Design Activities) was launched, which also served to test superconducting cavities in the SRF. The objective of IFMIF/EVEDA was ambitious. It consisted in producing a detailed, complete and fully integrated engineering design of the IFMIF device and validating the continuous and stable operation of the prototypes of each of its main subsystems.
The validation activities have been the subject of successful scientific collaboration between Japan and Europe through the Broader Approach Agreement in the field of fusion energy research. Thus, the design of the IFMIF during the EVEDA phase has been experimentally supported by the construction of three main prototypes:
- A Prototype Accelerator (LIPAc) at Rokkasho (Japan), fully representative of IFMIF’s low energy (9 MeV) accelerator (125 mA continuous wave deuteron beam).
- A lithium loop (ELTL) at Oarai (Japan), integrating all elements of the lithium target facility, complemented by corrosion experiments performed on the LIFUS6 lithium loop at Camugnano (Italy). Thus developing the diagnostic and purification systems necessary to show that the liquid lithium loop designed for IFMIF is viable.
- A high-flux test module and its internal components, which will be irradiated in a fission reactor and tested in the HELOKA helium loop in Karlsruhe (Germany), complemented by the creep fatigue test module, fabricated and tested at full scale in Villigen (Switzerland).
IFMIF-DONES
In parallel to the Broader Approach Agreement signed with Japan, the EU’s fusion pathway aims at producing electric power by the middle of this century. To this goal, the design and construction phase of DEMO has been accelerated by reducing the maximum neutron flux that its materials will have to withstand in the initial step. This significantly reduces the level of damage expected in them and, consequently, also reduces the requirements to be met by the neutron source to certify them. IFMIF can therefore be built in stages. The first of these, focused on DEMO requirements, is a project called IFMIF-DONES (DEMO oriented NEutron Source), launched in 2015. In addition to providing more relaxed technical specifications for its design, IFMIF-DONES will make it easier to spread the necessary investments over time.
IFMIF-DONES thus involves the construction of a somewhat more modest, but perfectly scalable neutron irradiation plant to fully crown IFMIF in the future in order to provide the information needed to design and build commercial fusion reactors.
In sum, the goal of IFMIF-DONES is to obtain the necessary experimental data on neutron damage to materials as soon as possible. This will provide sufficient engineering maturity to determine the technical specifications to be met by industry for the manufacture of components and the construction of buildings and auxiliary systems for DEMO design and assembly.
It should be noted that the IFMIF-DONES project has already made good progress in fixing its location in terms of safety, seismic, constructive requirements, etc. The Government of Spain and the Junta de Andalucía have made a formidable effort in this project. And the work of Croatia and other European partners has also been essential to promote this ambitious scientific and technological initiative. As a result of this collaborative work, no one now doubts that, if the plant is built, it will be located in Granada.