Seismic risk management in the IFMIF-DONES project
Earthquakes
There is no doubt that those of us who live on Earth perceive that our planet is moving. Its movements can be classified as external and internal. The most obvious of these are the rotation around the polar axis, which is responsible for the diurnal cycle, and the translation around the sun, which causes the annual seasons. Other external displacements, but less obvious, are the precession of the equinoxes and perihelion, nutation, the Chandler wobble and other orbital cycles. However, we will now focus on the internal ones.
Also called endogenous, these movements cause, among other effects, earthquakes, the intensity of which is measured by seismographs and accelerometers (instruments capable of detecting them even when their intensity is very low). In addition, these devices have allowed us to conjecture the internal structure of our planet, which we now assume to be basically made up of four concentric layers: a solid crust, a hot and viscous mantle, a liquid outer core and a solid inner core.
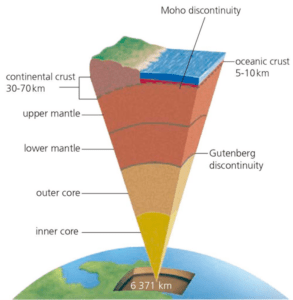
Earthquakes remind us, sometimes dramatically, that we live on a geologically active planet. And they happen frequently all over the world, but fortunately, most of them are imperceptible. Despite such frequency, they could not be explained acceptably until the last century thanks to Alfred Wegener, who provided evidence based on palaeotopography, climatology and the fossil record, underpinning the theory of continental drift and plate tectonics.
Indeed. The cold, solid crust of the Earth is fractured into multiple “continental plates”, like cyclopean pieces of a planetary puzzle that float on top of the much hotter and more fluid mantle and move continuously,[1] pushed by the enormous amount of matter that moves beneath them by convective effect (due to the thermal and density gradients between the deep and shallow parts of the mantle).
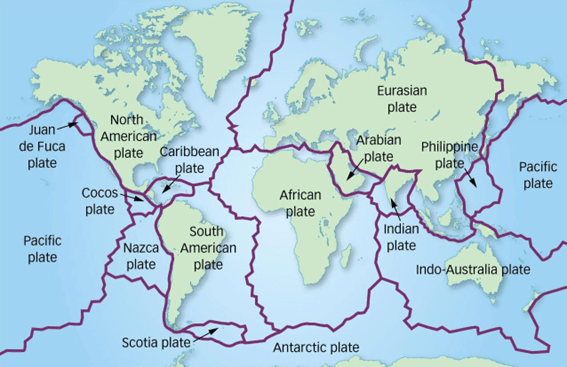
The continuous displacement of these tectonic plates generates stresses capable of cracking the earth’s crust and cause friction between their edges. If a certain threshold is exceeded, it can cause earthquakes, which can suddenly release an enormous amount of energy.
Seismic effects on ground and infrastructure
Historical and geological records warn us that earthquakes affect both human constructions and the ground on which they are erected, and can significantly damage them. This makes it advisable to analyse the construction of civil works from both geological and architectural perspectives in order to adapt their design to the “seismic scenario” and minimise possible damage during and after the earthquake. The aim is not only to achieve the best seismic-resistant construction but also to minimise the subsequent seismically induced effects (e.g. due to “liquefaction” of the ground when it is unconsolidated sedimentary, with a high water table).
Clearly, seismic dynamics are non-linear. Although this was already known in the middle of the last century, its implications did not emerge until the work of Seth Stein, after the great earthquakes of Sichuan (12 May 2008), Haiti (12 January 2010) and Tōhoku (11 March 2011). In fact, the latter, which caused the Fukushima accident, prompted the IAEA to start considering in nuclear power plant safety studies the risks associated with the subsequent geological effects, and not only those directly related to the earthquake itself [IAEA-TECDOC-1767 (2015)], calling for the use of palaeoseismology in risk prediction models.
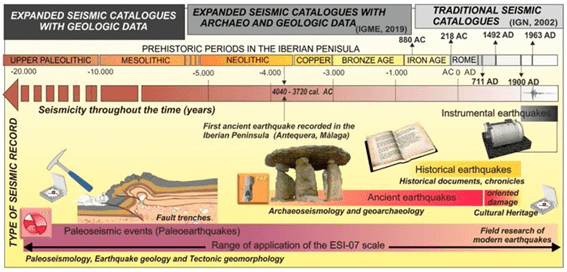
So, although statistical analysis is not enough to protect us from earthquakes, fortunately, geology, through palaeoseismological (using geomorphology, stratigraphy and structural geology), archaeoseismological (using archaeology and architecture) and instrumental seismicity techniques, can now anticipate, with remarkable accuracy, the where and how of an earthquake. And knowing where an earthquake is going to occur and how much energy it can release – with the help of the ESI-2007 scale – can mitigate many of the consequences.
IFMIF-DONES in the face of earthquakes
The Granada basin, formed by a group of Cenozoic sedimentary rocks, whose age ranges from the Neogene to the Quaternary, is located on the boundary between the Eurasian and African lithospheric plates,[2] more precisely on the boundary between the inner and outer zones of the Betica-Alboran mountain range, whose direction is NE-SW. Consequently, it is one of the most active seismotectonic areas of the Iberian Peninsula. In fact, earthquakes have been recorded in the area since 1431, when it suffered an intense earthquake that damaged the Alhambra Palace complex. Closer in time, at the end of the 19th century and also during the 20th century, earthquakes of various magnitudes (from 4 to 8 on the Richter scale) have been recorded until the beginning of 2021, when it was shaken by a seismic swarm, with earthquakes exceeding 4.4 on the Richter scale.
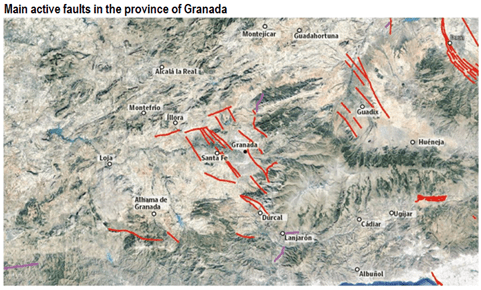
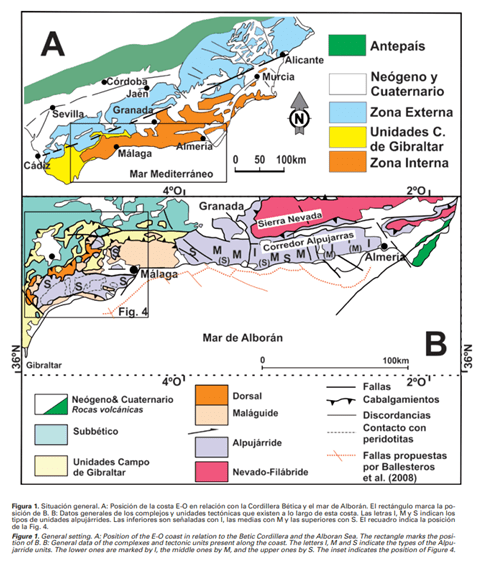
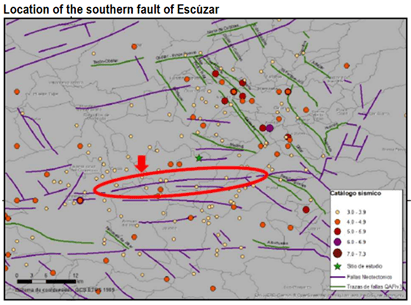
How does its proximity to the Escúzar fault influence the design and operation of the IFMIF-DONES facility?
The question is timely. The IFMIF-DONES project involves the construction of one of the most powerful deuteron accelerators in the world, whose main – but not only – objective is the analysis and qualification of materials for the construction of future fusion reactors. This device will be installed in the Metropolitan Park of Escúzar (Granada), on a 10-hectare site. The civil works will consist of a main building (which will house the accelerator, the lithium laces and the sample irradiation area) and around twenty auxiliary buildings. Focusing on the main building, its approximate dimensions are 160 metres long, 80 metres wide and 34 metres high.
In other words, IFMIF-DONES is essentially an instrument that projects subatomic particles over long distances with sub-millimetre precision (on the order of a human hair). A small tremor in the earth would be enough to throw it out of adjustment and cause it to lose its aim. In the first instance, if an earthquake were to occur during accelerator operation, the detectors would register a misalignment and shut it down safely. However, protecting such a delicate scientific instrument is very different from safeguarding the structural integrity of a bridge, a skyscraper or a hospital. How is this problem addressed in the IFMIF-DONES project?
Designing a resilient facility from a seismic perspective requires a thorough characterisation of the seismicity of the ground with all available scientific tools. An earthquake – like volcanism, hurricanes, tsunamis, the El Niño phenomenon, among others – is a non-anthropic threat, intimately associated with planetary dynamics, which, in the current scientific and technological state of humanity (still at rung zero of the Kardashov scale), cannot be prevented, which in no way means that on some occasions there cannot be some kind of control. Indeed. After a seismic assessment of the site, the design process considers two levels of protection: on the one hand, damage to people and equipment must be minimised in the case of the most intense earthquakes, as in any other building, and on the other hand, the operation of the facility must be ensured in the case of the much more frequent minor earthquakes.
Indeed, most of the endogenous movements, considering the protections to be put in place, hardly disturb similar laboratories in other parts of the world. A crucial feature of this type of facility is resilience, which prevents undue displacement as a result of normal day-to-day vibrations. This is achieved by seismic isolation devices and break points built into the connections between the machine and surrounding equipment to ensure that the device will remain stationary in the event of an earthquake.
Regarding the first level of protection, how to deal with the so-called maximum design earthquake, the strategy for designing IFMIF-DONES is to assume the acceleration parameters that a civil installation must withstand, according to seismic-resistant standards, but multiplied by a large safety factor. The anti-earthquake technologies envisaged are those used in similar scientific installations such as the ITER fusion reactor and other critical infrastructures. What do these considerations translate into?
Earthquake-resistant construction
It has already been said that earthquakes are the consequence of the sudden and remarkable displacement of tectonic plates, making regions close to faults or edges particularly vulnerable. Earthquakes generate omnidirectional shock waves that propagate through the ground at short, rapid intervals, causing lateral displacements for periods of up to minutes.
Indeed, a building structure is permanently subjected to the vertical forces due to its mass and the acceleration of gravity, which is very different from managing lateral seismic forces. In fact, when an infrastructure suffers an earthquake, the horizontal movement of the ground causes the building’s foundations to oscillate, causing walls, floors, columns, beams and their interconnections to vibrate. Very simplistically, it could be said that, due to inertia, the movement of the lower part of the building is different from that of the upper part, and this causes structural stresses, capable of breaking supports and causing the building to collapse.
Safety of building occupants
Of course, the first priority is always the safety of those in and around the building. Consequently, the design of the IFMIF-DONES project has taken into account earthquake-resistant structures and construction materials, thus minimising the likelihood that anyone inside or in the immediate vicinity of the building will be harmed by an earthquake.
Investment protection
Although less important than the protection of life, another priority is to ensure the integrity of IFMIF-DONES buildings.
How to build an earthquake-resistant structure?
We have seen that the IFMIF-DONES project will involve the construction of multiple buildings, each with a different typology and therefore a particular anti-seismic strategy. However, there are a few key considerations that have been taken into account in general terms.
Designing flexible foundations
One way to counteract the horizontal forces is to found the building by employing some technology that decouples the resonant frequency of the building from the typical earthquake frequency. One such method is to construct the building on flexible isolators (e.g. steel, rubber and lead pads), so that when the foundation moves during an earthquake, these isolators vibrate while the structure remains stable. This effectively helps to absorb seismic waves and prevents them from being distributed throughout the building.
Install vibration control devices
Most are aware of the usefulness of dampers in controlling undesirable oscillations in automobiles. The same philosophy has been adapted in buildings to reduce the amplitude of seismic shock waves and reduce stresses on the building structure. These are vibration control devices.
These devices are of different types. For example, dampers placed between the columns and beams at each level of a building, or ball-and-socket joints (a kind of pendulum which, when moving, rubs against a surface). In the event of an earthquake, the vibration energy is dissipated in the form of heat. The ball-joint technique is currently being studied at IFMIF-DONES.
“Transformational seismology” [3]
This innovative scientific discipline aims at achieving “seismic invisibility cloaks” capable of redirecting almost all seismic energy. Different procedures have been tested, for example, by creating a barrier with a hundred concentric rings of plastic and concrete buried under the building’s foundations. Thus, as seismic waves strike the rings, they travel more easily through the rings and are expelled outwards from this layer. As a result, the effects of the earthquake are channelled away from the building and dissipate into the ground.
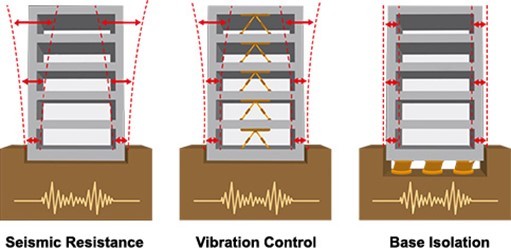
Building structural reinforcement
To prevent building collapse, seismic forces must be redistributed throughout the building structure. Therefore, shear walls, cross members, diaphragms and portal frames are essential.
- Shear walls, made of multiple panels, are usually supported by steel diagonal cross braces, capable of withstanding compressive and tensile forces. By counteracting these thrusts and pressures, the integrity of the building is maintained during the earthquake.
- Diaphragms, usually placed in the floor slabs, help to eliminate horizontal stresses by transferring them to the vertical structures of the building.
- Moment-resisting frames, by allowing columns, and beams to bend while the joints remain rigid, provide additional flexibility to the structure.
Earthquake-resistant materials
The selection of construction materials is undoubtedly a key factor in the design of IFMIF-DONES. For a material to withstand stresses and vibrations, it must be elastic, i.e. it must be able to tolerate large deformations and stresses. Structural steel, with its enormous variety of shapes, allows buildings to flex without breaking (wood is also a surprisingly elastic material, with great strength despite its light weight).
Innovative materials
Science and engineering are developing new building materials,[4] for example “memory” alloys, capable of withstanding high stresses and recovering their original shape. Fibre-reinforced plastic sheaths, for example, can give columns and pillars up to 38% extra strength and elasticity.
Consequently, the design of IFMIF-DONES has applied optimal seismic engineering solutions, suitably adapted to the local environment of Escúzar, thus ensuring a high degree of structural robustness and resilience to protect people and the technological infrastructure.
At IFMIF-DONES we continue to innovate.
[1] It may come as a surprise that one of the most accurate methods for measuring the displacement of continental plates lies in the observation of quasars. More than two hundred thousand such astronomical objects are known, all of them so distant from the Earth (the nearest is 780 million light years away and the furthest is 13 billion light years away) that their proper motions are undetectable. Thus, when an astronomical observatory sees any change in its position, it can only be interpreted as the motion of the continental plate on which the observatory sits. It has thus been found that continental plates usually move at speeds of only a few centimeters per year. During an earthquake, on the other hand, this displacement is much greater.
[2] The movement of these plates, in addition to bringing Africa and Europe closer together by four or five millimetres a year, also causes several hundred earthquakes every year, most of them imperceptible.
[3] At the end of the last century, a new discipline called transformation optics emerged, which studies the possibility of rendering objects invisible to electromagnetic waves, for example, by looking for materials with negative refractive indices.
The analogies between electromagnetic and seismic waves (replace the terms electrical permittivity and magnetic permeability by, respectively, density and modulus of elasticity of the soil) were soon realised, and efforts are being made to transfer the knowledge gained in the field of transformation optics to seismology.
[4] Materials science is investigating the properties of some natural substances to reinforce the structure of buildings. For example, applications are being sought for the adhesive but rigid fibres of mussels or the silk of arachnids. Bamboo and 3D printed materials, on the other hand, make it possible to create lightweight interlocking structures without shape limitations, which can give buildings greater strength.