NEAR NUCLEAR FUSION. How to contain plasma to prevent its escape
In another article it was already noted that nuclear fusion reactions release energy (in fact our Sun is a giant fusion reactor with lighter elements colliding with each other, transforming into heavier elements and releasing a colossal amount of energy). Harnessing this energy, which is virtually inexhaustible, far cleaner in terms of harmful waste and very reliable for generating electricity, has been one of the most ambitious goals since the 1940s. But controlling nuclear fusion is not technically simple.
We also saw in the same article that one of today’s most promising fusion reactions is that of deuterium and tritium. Its achievement requires confining these reagents with the adequate density and for a sufficient time, at a temperature over 100 million degrees Kelvin, in a plasma state. No material is capable of withstanding such conditions! How, then, can we build a container for this plasma?
Bottling plasma
Let’s remember that, since plasma is a gas formed by electrically charged particles, an ideal approach is to confine it by means of magnetic interactions. However, attaining a magnetic field capable of effectively confining plasma is a great challenge for controlling nuclear fusion.
How can we prevent the highly energetic particles in plasma from leaking from the reactor? It is well known that, within magnetic fields, charged particles follow curved trajectories. We must therefore ensure that the magnetic force acting on the charged particles in plasma stably balances the force with which they tend to escape.
Due to its higher degree of development, we will focus on the tokamak, a type of closed magnetic configuration in the shape of a doughnut (mathematicians call these shapes toroids).
There are other concepts of toroidal containment with operational advantages over the tokamak. Three of them are:
● The stellarator, similar to the tokamak, but whose magnetic field is produced by external coils. In this case the plasma current is essentially zero and, therefore, the problems inherent in maintaining a high plasma current are avoided.
● The reversed field pinch, which differs from the tokamak in that it operates with a weak toroidal magnetic field. The result is a compact, high power density reactor with copper coils (instead of superconductors).
● The so-called compact toroid, which is a “doughnut” deprived of a hole in the centre and therefore without some engineering complications.
The tokamak
In the mid-1960s, Soviet physicists studying plasma introduced toroidal confinement. In fact, tokamak is an acronym derived from the Russian (toroidal’naya kamera s magnitnymi katushkami) for the so-called “toroidal magnetic confinement”, which is currently the most common magnetic containment scheme. The fact that plasma adopts the form of a doughnut without altering this shape is due to a system of magnetic fields whose lines of force follow two directions: a poloidal and a toroidal one, as seen in Figure 1.
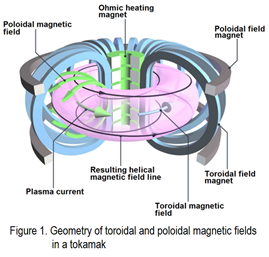
The combination of magnetic fields, toroidal (in blue) and poloidal (in green), results in a magnetic field with a vertical helix shape (in black). The resulting forces of that combined field make the plasma particles trajectories also horizontal helixes (“doughnut” type geometry), as shown in Figure 2.

In addition, it should be noted that, in order not to lose energy quickly when its particles collide with air molecules, the plasma must be inside a vacuum chamber, also obviously in the shape of a doughnut.
Heating plasma
It has already been said that in order to produce nuclear fusion reactions plasma must be heated over 100 MK. Undoubtedly, the combined effect of the magnetic fields used to confine plasma help to heat it, but it is not enough. Alternative forms of heating are required, and two methods have been developed for this purpose:
● Heating by electromagnetic waves (whose spectrum ranges from radio frequencies to microwaves). Due to its nature, this type of wave propagates easily in plasma. The absorption of energy usually depends on a resonant interaction between the wave and plasma.
● Neutral beam injection heating. It consists of bombarding the plasma with neutral particles. The collision between both ionizes those particles. The confining magnetic field prevents their exit, and the ionized neutral particles can transfer most of their energy to plasma.
Magnetic confinement today
Several decades of research on nuclear fusion have produced two kinds of success. On the one hand, current knowledge about plasma physics already allows a quantitative assessment of many aspects of fusion reactors. And on the other hand, what is eventually most interesting from an industrial perspective, the control of plasma has brought the experiments to a point where the fusion energy produced equals the energy necessary to create and maintain plasma.

Several plasma experiments have been conducted to solve the problems of fusion, but three stand out:
● The Joint European Torus (JET), located in Culham, UK, is currently the largest tokamak in the world, and operates on the basis of the European Fusion Development Agreement (EFDA).
● The Japan Torus-60 (JT-60), located in Naka, Japan, is a tokamak-type reactor, the flagship of Japan’s nuclear fusion research programme.
● The Tokamak Fusion Test Reactor (TFTR), located in Princeton (USA).
The construction of the International Thermonuclear Experimental Reactor (ITER) currently ongoing in Cadarache (France) is the next major step. This device will make it possible to investigate not only the physics of an ignited plasma, but also reactor technology itself and the development of new materials, capable, for instance, of withstanding intense neutron bombardment.