On the edge of electricity production by nuclear fusion
THE POST-ITER ERA
The ITER programme (acronym for International Thermonuclear Experimental Reactor), which is the result of several decades of research in plasma physics and fusion reactor technology, is also the culmination of an international collaboration that could end just as soon as that stage is completed. Although for the ITER partners the construction of the DEMO (DEMOnstration Power Plant) is the accepted next step, China, the European Union, India, Japan, Korea, Russia and, to a lesser extent, the United States have already expressed different views on the specifications of a post-ITER demonstration reactor (see table below). As an upshot, there are numerous competing projects for the future, but the exact details of which, in any case, require analysis of the ITER experimental reactor’s operation.

What is beyond all doubt is that, driven by the European promoters of ITER and Japan, DEMO is the main post-ITER project.
THE DEMO PROJECT

With the ITER experimental reactor learning, the following step will be possible: DEMO, which to a certain scope is more than a machine, it is a process. This project consists of fine-tuning the technology to design and build a demonstration nuclear fusion reactor to produce electricity by 2040 and bring fusion to the threshold of its industrialisation. Consequently, DEMO requires a comprehensive consideration of all the physical and technological requirements and their compatibility with their respective boundary conditions.
From a physics perspective, the challenges are manifold: ignition will be performed with a denser and more pressurised plasma, but always taking care of the container walls integrity. And, in terms of technology, there is a need to develop robust wall materials, high temperature coolants and energy efficient methods of igniting the plasma and maintaining its current. Thus, multiple areas of research are involved:
- Continuous or long-pulse operation.
- Process with high-density plasmas.
- Plasma-wall interface to minimize plasma cooling.
- Diagnostics and control of plasmas.
- Plasma current switching and actuation.
- Materials for structural and functional components.
- Container and coolant loop components.
- Tritium cycle.
- Vacuum technology.
- Superconducting technology.
- Remote control and maintenance techniques.
- Efficiency, reliability and availability of all systems.
FEASIBILITY OF DEMO
DEMO will show that it is possible to produce electricity using the energy of thermonuclear fusion reactions that occur within a high-temperature heated plasma (∿100 MK).
For such a project to be viable, three minimum requirements are to be met:
- The fusion reactions have to income more energy than is needed to keep the plasma “burning” (plasma capable of producing fusion reactions within it).
- It must be possible to convert the energy produced by the fusion reactions into electricity.
- Fusion reactions must be maintained over long periods of time to produce electricity indefinitely.
As for the first condition, the tokamak JET (acronym for Joint European Torus) has today the record of production, but the performance rests negative, as it generates only 65% of the activation energy consumed. Showing that a positive energy balance is possible is one of the points of ITER, with which it is intended to produce ten times the total energy consumed.
With regard to the second constraint, it should be noted that during fusion reactions energy mainly comes in the form of gamma radiation. This type of energy is difficult to ‘recover’, in particular through heat exchange with the help of a cooling fluid. To overcome this major obstacle, several experiments are currently being conducted with different energy transfer systems.
However, the greatest challenge to date lies in the third obstacle. Indeed, a commercial power plant, except for maintenance reasons, has to be able to produce electricity without interruption over long periods of time. Today, nuclear fusion tests are far from this achievement, as the devices are only able to keep the plasma burning for a very short time. The record of 390 seconds is held by the tokamak Tore Supra (current WEST project). ITER’s ambition is to maintain a burning plasma at least 400 seconds (the simulation models indicate that, beyond this time interval, providing external energy would no longer be necessary because the plasma would already self-sustain the fusion reactions).
It is obvious that the exact characteristics of DEMO will be designed on the ITER results basis, which will have to be analysed very carefully. Therefore, these specifications cannot be defined at present. On the contrary, there are more questions than answers: what materials should be used in the device, in particular where the magnetic intensity is highest in a continuously operating reactor? [1] Which systems will be more efficient in ‘recovering’ energy from gamma radiation and in capturing high-energy neutrons? Which fuels will allow the long-term operation of a fusion reactor in the future? Which design should be adopted by DEMO? [2]
SOME TECHNICAL CONSIDERATIONS
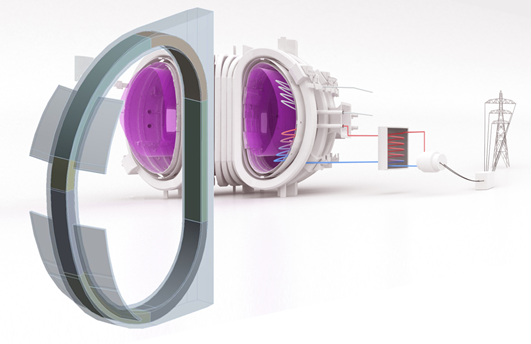
In this papers series it has been repeated that the candidate fuels for first generation fusion reactors are deuterium and tritium (both isotopes of hydrogen). During the fusion reaction, the nuclei of these two elements join together to form helium, emitting an enormous amount of energy. We also know that these nuclei, in order to join together, must come within 1 femtometer of each other. At that distance, the quantum ‘tunnel’ effect allows them to overcome the coulomb repulsion barrier (do not forget that both nuclei are positively charged).
That is why, in addition to the high density of the plasma, the activation energy is so important in nuclear fusion reactions, with temperatures of about 100 MK. This is achieved, remember, using different energy sources, such as ohmic heating (electrical currents induced in the plasma), microwaves, ion beams or injection of neutral beams.
Together with helium, fusion reactions produce ultra-high-speed neutrons and high-energy electromagnetic radiation. This extraordinarily hot helium will compensate for losses that would end up cooling the plasma very quickly. But, in addition, the tokamak’s containment vessel will have a coating (ceramic or some kind of composite) inside which some tubes accurately arranged will conduct liquid lithium. The capacity of this alkali to absorb neutrons, originating helium [3] and tritium, satisfies two objectives: to serve as the first cooling loop and to regenerate the tritium necessary for the fusion reaction. Let’s say that during this regeneration process the amount of lithium consumed is very tiny.
The heat absorbed by the lithium from this first circuit is transferred to another cooling loop, through which pressurized liquid water will probably circulate. The thermal energy accumulated in this secondary loop will be used to generate water steam in a heat exchanger, which will be used to drive the turbines of an electric power generator.
Thus, due to the scarcity of tritium, the candidate fuels for DEMO are deuterium and lithium (the precursor element of tritium, as seen above). Precisely, another of ITER’s main objectives is to test the partial likelihood of this lithium-tritium conversion cycle. Consequently, DEMO will be the machine where the whole of this cycle will be investigated before building the industrial prototype fusion reactor.
BRIEF COMPARISON OF DEMO WITH ITER
If producing 500 MW during 400 seconds and multiplying tenfold the energy needed to start the reaction is the goal of ITER, the goal of DEMO is to continuously produce four times the power achieved in ITER (2 GW), multiplying by twenty-five the energy needed to start the reaction. In short, DEMO should achieve a production similar to that of current power plants.
DEMO is therefore expected to be the first fusion reactor to supply electricity to the grid in a commercially viable way (the previous experimental devices, such as ITER, mainly generate thermal energy which is dissipated into the atmosphere as water vapour). In sum, the main goals of DEMO are the extrapolation of continuous or quasi-continuous (steady-state) operation, the study of efficient power generation systems, achieving a ratio between generated and consumed power (Q) of 30 to 50 (compared to Q ≥ 10 for ITER), and the regeneration of tritium.
To reach these objectives, the DEMO tokamak will require an outer diameter of about six to ten meters, and a 30% denser plasma. However, its construction is likely to be considerably simpler than that of ITER, since the design will focus on power generation rather than on exploration of the plasma regime, and hence there will be far fewer diagnostic systems.
DEMO WILL GENERATE LITTLE WASTE AND MINIMALLY HAZARDOUS
The products of the DEMO’s fusion reaction are not radioactive, but the metal parts of the plasma containment vessel are neutronically activated. However, this radioactivity dissipates within a few decades (the half-life of this residue compared to that produced in nuclear fission is negligible). Therefore, the associated risks are much lower.
AND AFTER DEMO?
The DEMO project will pave the way for the commercialisation of the first fusion reactors, the cost of which should be less than a quarter of the DEMO price. As they are unlikely to come into operation before 2040, this implies starting to build DEMO before ITER (the first shot of which is scheduled for December 2035) has reached its full potential. In any case, the following stages will be the result of free political choice in each country concerned. Conversely, it is reasonable to expect that fusion power generation will become a reality in the second half of this century.
[1] To answer this question and develop advanced materials, the IFMIF-DONES project was established, which we will discuss at length in this papers series.
[2] During the ITER construction and the DEMO design, other facilities will be operated to study the regeneration of tritium, and the removal of heat and its transformation into electricity, etc.
[3] This noble gas, which can be considered the “slag” of this combustion, will have to be removed to avoid its accumulation.